PHYS 115 Ad Hoc Project
Describing Neuron Functions Through Physics
Making Comparisons
You may have already begun to notice some similarities between the physics and neuroscience sections prior to this page. In order to solidify these points and make the following sections clearer, try to convince yourself of the following comparisons:
-
Ions within a cell can be modeled as point charges from physics. Although this is not exactly true as ions also have additional forces and mass, the concepts of charge interactions remain nearly identical. Just like positive charges repel each other, a Na+ and K+ ion will be repulsive to each other as well.
-
The EMF of a battery is equivalent the Vm, or the membrane potential of the cell. Just like how a battery raises the potential of electrons that pass through it, the sodium potassium pump pushes ions towards locations opposite to where their concentration gradient is leading to.
​
When these different concentrations of ions move from a higher concentration to a lower concentration, they lose energy just like a particle moving down an electric field. This force is called the concentration gradient, which can be in the same or opposite direction as the electric force of the ions to create the electrochemical gradient. This determines the overall movement of ions within the cell membrane.
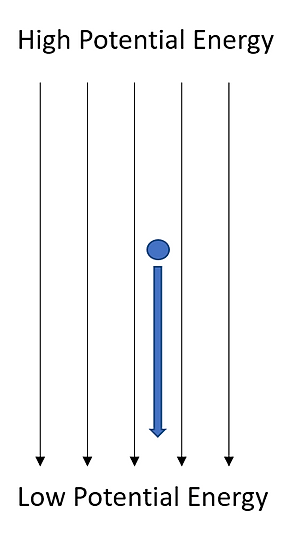
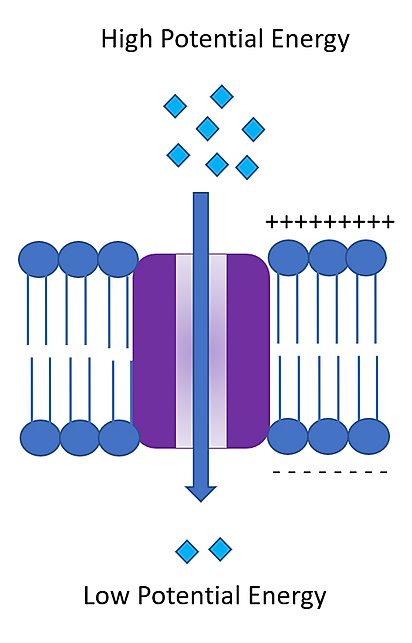
Modeling an Action Potential Through an RC Circuit
As we learned before, an RC circuit is characterized by a specific resistance and capacitance. However, you may be surprised to know that a neuron follows the same concept of an RC circuit. Ions can only move through the membrane at a certain rate, and when they can’t move through any channels, they still feel the electric forces coming from the opposite side of the membrane. As such, within a neuron:
-
Resistance is inversely proportional to the number of ion channels open (more ion channels open = lower resistance​)​​​​
-
Capacitance is proportional to the surface area of the cell membrane (more area = more ions can push against the surface = more charge stored)
​
When an action potential occurs, Na+ ions have two choices of where to go: through the ion channels (resistor) or line up against the membrane due to charge attractions (capacitor).
According to Kirchhoff’s Current Law, current will always flow through the path of least resistance. In an RC circuit, this is always charging the capacitor, or in our neuron model, it would be ions forming an “ionic sheet” against the cellular membrane that could later be discharged. However, as ions fill the capacitor, there is stronger repulsion forces among the like charges on one side, so ions will begin to move through the ion channel instead despite its resistance.
​
According to Kirchhoff’s Junction Law, both the currents going into the capacitor and going through the resistor must add to the total current within the circuit. As such, we know that as less ions stack against the cellular membrane, more ions move through the ion channels, leading to a depolarization.
Understanding the Difference Between a “Signal” and a “Current”

You may have noticed that I always referred to APs throughout this website as “electric signals” rather than “electric currents.” This is a very important distinction and a common misconception. As noted before, Kirchoff’s loop law tells us that every complete loop with a constant resistance within a circuit has a total potential change of zero. As such, the current must be the same across the entire loop using Ohm’s law, V = IR, where V and R are constant.
​
When a neuron fires an action potential, there is not a constant current across the length of the entire axon. Instead, there are momentary local changes in membrane potential across the axon. As such, this is a signal, and not a current. Intuitively, this should make sense because a neuron does not actually create a circuit; the same AP that was produced never returns to the cell it was initiated from.
​
But we said earlier that a neuron acts as an RC circuit, right? This is because the RC circuit model of a neuron is only applicable to a small subsection of the membrane in the moment that an action potential is occurring. In this moment, charge is flowing from one side to another. Even then, due to the vast variability of biological systems compared to idealized physical models, this model will never be perfectly correct.

The Na+ channel (left) and the K+ channel (right) function similarly to one another, but have opposite directions that both act as an RC circuit. The Na+ channels open first, causing a depolarization (more positive Vm) in the cell, followed by the K+ channels opening, causing a repolarization (more negative Vm) in the cell.

Going Further!
Unfortunately, I am unable to cover all of the vast topics within the intersection of neuroscience and physics. However, I highly encourage you to explore this topic on your own and even consider what applications you might be able to discover yourself! Here are some potential resources to consider:
​
Clinical Applications
-
Depolarization in the heart and electrical signals that act independently from the brain - The Heart's "Little Brain" (jefferson.edu)
-
Seizures caused by “electrical storms” in brain - The Neurobiology and Consequences of Epilepsy in the Developing Brain | Pediatric Research (nature.com)
-
EEGs - Although not a (csulb.edu)
​
Other Applications
-
Quantum physics in neuroscience - Quantum physics in neuroscience and psychology: a neurophysical model of mind–brain interaction - PMC (nih.gov)
-
Lab practices such as fiber photometry - Frontiers | Simultaneous Electrophysiology and Fiber Photometry in Freely Behaving Mice (frontiersin.org)
-
Neurons in pigeons linked to inner ear activated by magnetic fields - Neurons in a pigeon’s brain respond to magnetic fields (nationalgeographic.com)